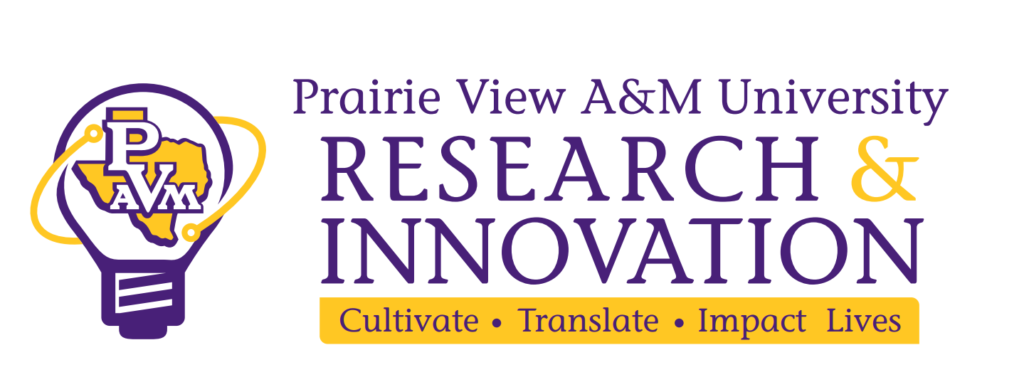
The Vice President for Research and Innovation has established a new initiative Faculty-Research and Innovation Success and Excellence (Faculty-RISE), that strategically invests in high-priority areas of research and innovation clusters identified for the university. The Faculty-RISE program will provide needed support in disciplines that can lead to the formation of renowned scholarly and research centers and institutes. Faculty-RISE will serve to push the boundaries of knowledge and be critical points of convergence for the university and cultural experience for students and faculty, encouraging multi- and inter-disciplinary collaboration. Listed here are selected projects supported by the VPRI Faculty-RISE program. To see more details about the corresponding projects, please visit the faculty in their respective laboratory, department, or college websites.
High-Pressure Combustion of Liquid Fuels in Microgravity
Dr. Yuhao Xu, Assistant Professor, Mechanical Engineering, College of Engineering
This project will support the initial effort of developing a ground-based research program to investigate the droplet combustion of liquid fuels at elevated pressures in a microgravity environment that promotes spherical symmetry. The relevance of the project stems from the fact that petroleum-based liquid fuels are currently used to power combustion engines such as diesel engines and gas turbines. In these systems, the operating pressure (up to 60 atm) could exceed the critical pressure of the liquid fuel injected. Consequently, droplets in the spray often evaporate and burn in ambient air at pressures and temperatures well above the critical state of the fuel. However, there is very little fundamental information available to understand the combustion of liquid fuels at these elevated pressures. The microgravity environment achieved in a drop tower facility provides the opportunity to reveal the fundamental information of liquid fuel combustion at high pressures that is not currently available. At low gravity, buoyancy-induced flows are eliminated. What remains is often a transport symmetry that significantly facilitates numerical modeling of the phenomena. Therefore, this project aims to develop a ground-based program to study droplet combustion of liquid fuels at elevated pressures in microgravity that promotes spherical symmetry.
Develop Novel Tissue Engineering Materials for Regenerative Medicine, Cancer and Radiobiology Research via Interdepartmental Collaborations at Prairie View A&M University
Yunxiang Gao, Assistant Professor, Department of Chemistry, College of Arts and Science
This project aims to develop novel soft materials for several frontier applications in modern smart materials engineering and tissue engineering fields. Deeply merged macromolecular science and nanotechnology will be the core of this research. Liquid crystalline elastomers (LCEs), the macromolecular part of the merged field, is a type of novel soft matter formed by aligning and crosslinking one-dimensional rigid liquid-crystal molecules using elastic segments. LCEs have two unique features compared to other traditional soft matter: programmable shape-memory-transitions under controlled stimuli and crystalline-structure-induced anisotropic properties. Through re-innovating and optimizing the synthesis of LCEs or merging LCEs with specific nanomaterials, we will endow LCEs with fascinating new functions that they never had before as conventional macromolecular materials. LCEs may have ultra-fast actuation, radio-screening, and programmable tissue engineering control. First, we will embed photothermic and radio-screening nanomaterials in LCEs to resemble artificial muscles offering rapid actuation in soft robotics applications. Second, we will further use the material obtained to improve tissue recovery under high-dosage radio-exposures during space missions. Third, we will try to develop a 3D tissue engineering scaffold that can self-grow over time with the growth of live tissues. Samples can also be provided to cancer researchers as in vitro tumor culture platforms.
Impact of an External Vascular Access Device on Arteriovenous Fistula Patency Rates
Dr. April Lovelady, Assistant Professor, Department of Mechanical Engineering, College of Engineering.
According to the United States Renal Data System, the number of patients in need of hemodialysis is growing by 4-6% annually. The devastation of end-stage renal disease (ESRD) affects more than 703,000 Americans, with over 124,000 newly-diagnosed patients each year. Vascular access methods for hemodialysis have traditionally been plagued by a variety of complications. The most common method of access is the arteriovenous fistula (AVF), which is created by using a patient’s natural vasculature. While hospitals and dialysis centers alike are incentivized by Medicare to utilize AVFs for venous access, more than half of fistulas never mature to become useable.
Furthermore, due to blockage or narrowing, a functioning fistula fails every three years on average, rendering them useless. These issues can be addressed through an innovative vascular access device designed to surround the vein, integrating with the nearby soft tissues to reinforce the strength of the vessel while guiding the needle to a precise location within the vessel’s lumen. This project seeks to assess tissue integration into and around the vascular implant and evaluate the histologic response of an arterialized vein surrounded by an external, rigid, porous device. Further, this project will normalize the independent cannulator effect and show long term survival (patency) of the AVF model when supported externally by the Ark.
Thiamin and Thiamin Analogues as Carriers for Drug Delivery to Cancer Cells
Dr. Sameh Abdelwahed, Assistant Professor, Department of Chemistry, College of Arts and Sciences
Thiamin is an essential cofactor in all cell types. It is critical for the activity of many vital enzymes in cellular metabolism, pyruvate dehydrogenase, alpha-ketoglutarate dehydrogenase, transketolase. Cancer cells require metabolism to tolerate their survival, and a corresponding 2-fold increase in thiamin transport was observed in the tumor cell, suggesting a significantly increased requirement for thiamin during hypoxic stress. Most anticancer drugs in clinical are limited by their toxicity, and many of these compounds still lack tumor selectivity and have not been therapeutically useful. For example, although, dichloroacetic acid (DCA) has been studied as a potential anticancer drug because it inhibits the enzyme pyruvate dehydrogenase kinase, an enzyme which plays an important role in carbohydrate metabolism, DCA is limited by its toxicity to normal rapidly growing cells. My approach, to enhance the specificity of DCA may be to exploit the upregulation of thiamin transport via the conjugation of DCA with thiamin molecules affording enhanced cancer cell-specific uptake. Our studies will not be limited to DCA, but it will include a variety of anticancer small molecules, and use thiamin as a drug delivery vehicle.